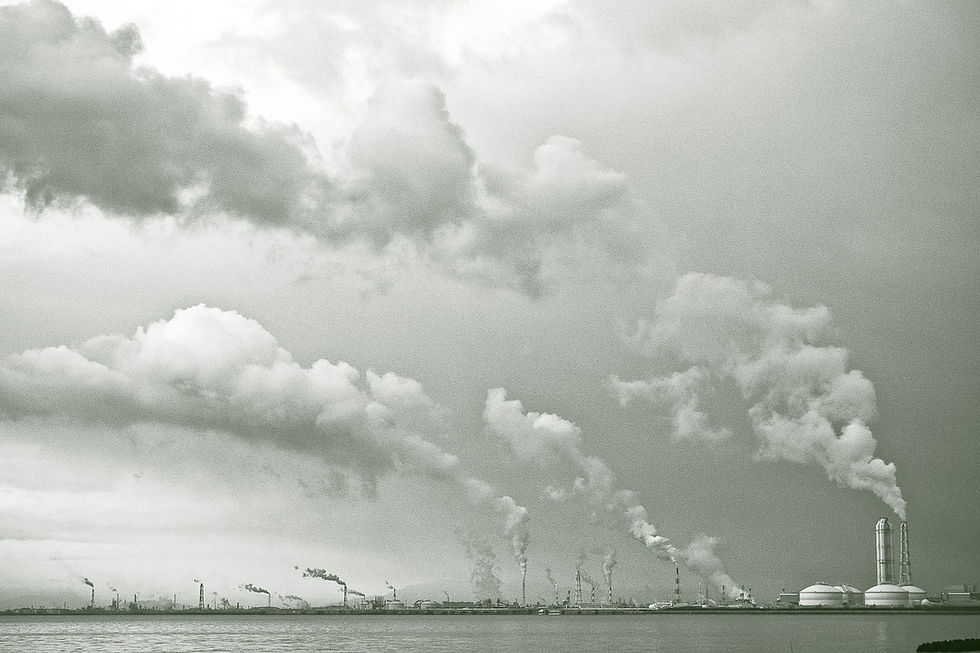
Captured carbon dioxide has and can be put into just about anything. Vodka. Activewear. Perfume. Jet fuel. Laundry detergent. Shower soap. Sneakers. Straws, forks, spoons, and knives. Concrete. Nanotubes. Bioplastics. Steaks, salmon filets, and chicken breasts. Watches. Pen ink. Party dresses. Vegan leather. Hand sanitizer. Sunglass frames, handbags, and tote bags. Aquaculture fish food. Diamonds. The list goes on and on -- including, most recently, a plan from Coca-Cola with to turn captured CO2 into sugar.
The number of uses for captured carbon dioxide is constantly growing. And the possibilities are endless, according to Merritt Dailey, P.h.D. candidate at Arizona State University’s Center for Negative Emissions. “There are countless chemical pathways to utilize carbon and a myriad of potential products,” she said. “There is no one way to do it.”
Turning carbon into products via carbon dioxide removal or CDR is better known as carbon capture utilization or CCU. The process generally relies on one of two methods of CDR, using either traditional carbon capture (securing gasses from industrial processes) or direct air capture (DAC), securing carbon from ambient air.
In 2019, as the market for upcycled carbon products began to emerge in force, Vox reported that by 2030, it could be a $1 trillion market, topping that of solar PV and wind. Now, estimates show that between this year and 2031, the market for both carbon utilization and storage will grow by over 19% annually.
While the list of carbon-containing products is long, it’s not nearly as vast as the amount of CO2 in our air. And it might lead one to wonder: how much can something like upcycled-carbon-infused sugar actually help the planet?
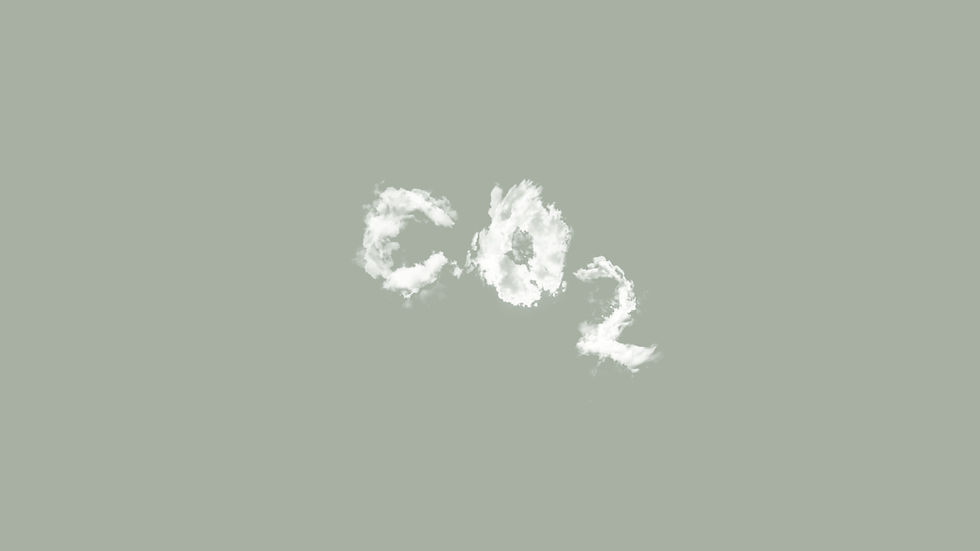
Before we tackle that atomically massive question, we must first understand why companies are making carbon a commodity. The short answer, as Dailey put it, is to make carbon into something people will pay for, create a market for it, and drive down adoption and technological learning to make carbon capture cheaper.
DAC is extremely expensive. But, as put by the Direct Air Capture Coalition and the Intergovernmental Panel on Climate Change (IPCC), there is no time to waste in the push to permanently remove CO2.
In order to keep the temperature rise within 1.5 degrees Celsius, as outlined in the Paris Climate Agreement, we have to reach net-zero emissions by the infamous year of 2050 and the halfway point by 2035.
Strategies that reduce emissions such as renewable energy alternatives and energy efficiency likely aren’t enough on their own. So it’s commonly accepted that CDR must be a part of the equation. The controversy lies in the question of how much CDR is required, and if the controversial dream of negative emissions stagnates our mitigation of greenhouse gas emissions.
Nevertheless, most models used by the IPCC estimate that carbon capture will account for 14-20 percent of this mitigation challenge. The traditional model of carbon capture and sequestration (CCS) treats carbon like we treat hazardous waste products, burying gigatons and gigatons of it deep underground.
If carbon is buried underground, we can have a very high degree of confidence that it will stay out of the atmosphere for millennia, which Dailey says, is what we need to stop climate change altogether.
The price of capture varies depending on the industry and category of technology. In the case of DAC, it’s steep: ranging from $250 to $600 a ton. Depending on market development, that price tag could drop to $150-$200 a ton over the next 5-10 years. Initiatives by the Department of Energy may even lower it to $100 a ton.
CSS on the other hand is less expensive. According to a Harvard policy brief, the shallow end of the spectrum averages around $30 per ton, and the deep end, around $120 per ton.
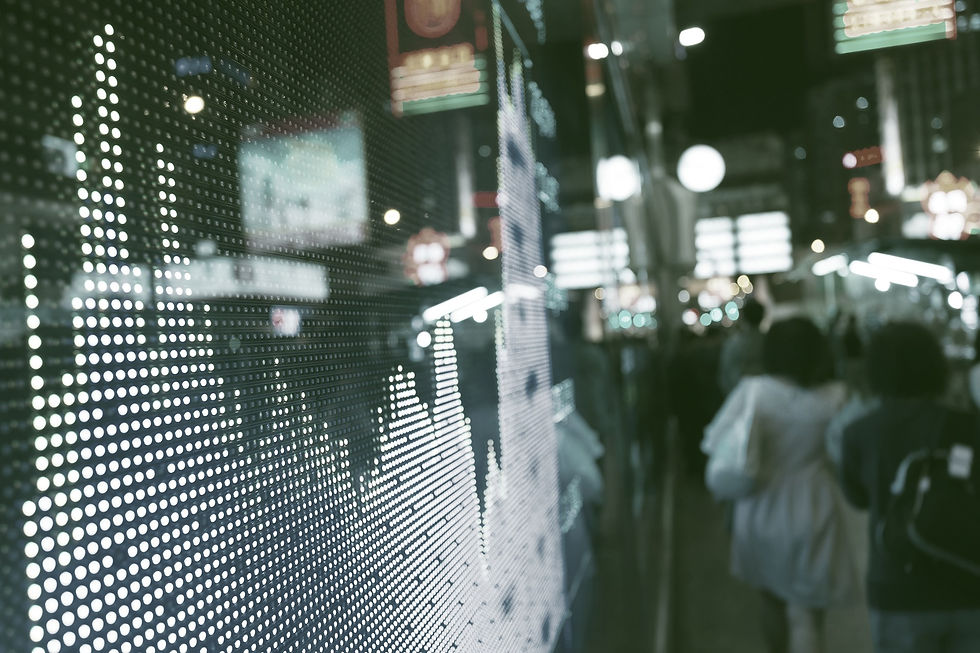
The process of CCS is used to decarbonize hard-to-electrify industries like coal, gas, cement, and steel production, where industrial emitters are highly location specific and CO2 concentrations are high. DAC on the other hand uses a series of chemical reactions to pull CO2 from the air and typically, injects it into rock formations buried deep below the Earth’s surface.
The reason DAC is much more expensive than CCS is mainly because of the level of CO2 concentration it deals with.
When we burn fossil fuels, releasing carbon into the air, we increase the parts per million (ppm) of carbon dioxide that exists in our atmosphere. Over the past 100 years, these levels have gone sky high, currently around 417 ppm. The concentration of CO2 emitted by coal plant exhausts, for example, is much higher, inflating that of the atmosphere 300 times over.
According to the Climate Portal at the Massachusetts Institute of Technology, from an engineering perspective, it's easier to capture carbon from a gas with a higher concentration of CO2 because there are more molecules to grab. However, even if CCS could remove 99% of the CO2 emitted by a coal plant exhaust, the CO2 concentration left behind is still equal to or higher than that in the atmosphere.
Thus, DAC picks up where CCS leaves off in the carbon capture triathlon, grabbing the baton for the hardest event: capturing carbon at a lower concentration. Because the chemical task is harder, this makes DAC much more expensive Dailey says.
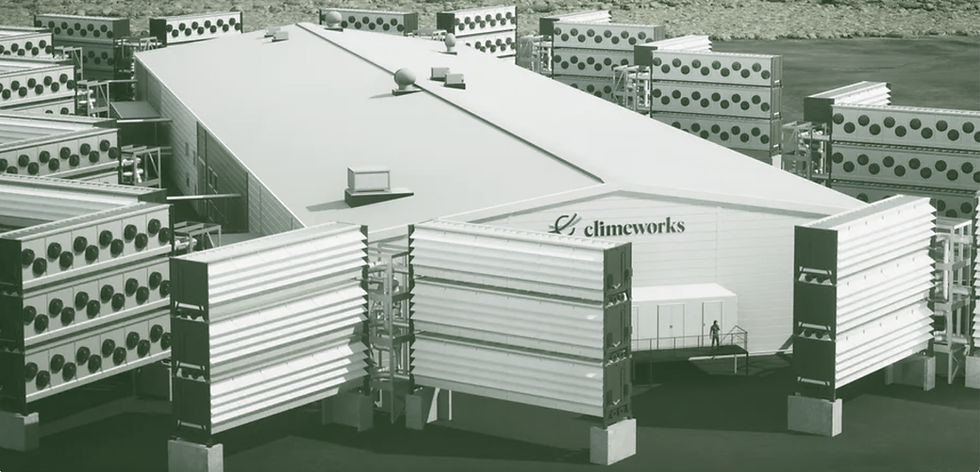
It requires specialized equipment, and more energy, time, and money. For 650,000 years prior to humans, CO2 ppm didn’t go over 350. And when many scientists think this level is much healthier for the planet, CCS, DAC, and low-carbon efforts have a lot of work to do. How much work exactly? 10 billion metric tons of work annually until 2050, with an increased 20 billion removal capacity by 2100 according to the World Resources Insitute. In terms of reaching those goals, we’re not even close.
Notably, carbon capture utilization products don’t meet our carbon burial needs. Not by a long shot. Storing carbon for millennia is what will mitigate climate change. If putting carbon in jet fuel, for example, just puts it back in the atmosphere, one might ask, why CCU is necessary at all.
As Dailey explains, if CO2 is made into vodka or jet fuel, that CO2 will be re-released into the atmosphere upon use. While it is not carbon negative, CCU is a helpful tool in the decarbonization toolbox. Most of these tools are pretty costly. Thus, the main driver for CCU is the demand. It bets that carbon vodka and cement, for example, can help scale up and cheapen the CDR process.
The Inflation Reduction Act represents the first time the U.S. has funded the technological removal of carbon. It’s expected to jumpstart the industry.
“There’s a growing awareness that climate change is real. It’s pressing. We’re feeling the effects now, and if you can create a market for carbon dioxide, it incentivizes capturing it,” Dailey said. “That means less carbon would be emitted directly into the atmosphere, and if it is emitted then someone has an incentive to draw it down.”

Capturing, reusing, and sequestering enough carbon to bring the atmosphere into balance is one example of a ‘circular economy.’ Depending on who you ask, large-scale CO2 recycling could partially close the carbon loop, and make a circular economy a reality.
Unfortunately, all CCU isn’t as cyclical. At present, the majority of CCU is used for enhanced oil recovery (EOR). In EOR, pressurized CO2 is injected into existing oil and gas reservoirs to squeeze out more gas than stored. (Think the petroleum industry.) It monetizes C02 by enhancing the amount of oil produced, allowing carbon to reach an appreciable scale.
This sets EOR apart from other CCU ventures because it's the only carbon sequestration industry of scale. Nevertheless, the environmental benefits of EOR are thorny. While it reduces the carbon intensity of oil, the subsidization does not lessen oil and gas use. Quite the opposite, it increases it. And while most of the CO2 stays underground and it can help drive down the cost of CSS and DAC, the sources of dispute lie in the likeliness of it reaching carbon negativity and the fact that it can keep polluting fossil fuel facilities alive. Not to mention that many operations don’t use anthropogenic CO2, or rather carbon produced by us, and are thinly regulated. (Vox has a great explainer on the EOR debate.)
Putting captured carbon into consumer products is less environmentally thorny. Many operations of these products use renewables to avoid emitting more carbon. But, it’s important to note that not all do.
“Plenty of people will claim that their product is carbon neutral or carbon negative,” Dailey says, “ but unless you do a really rigorous look at how it's produced — called a lifecycle assessment — some of these claims will be proven false. There’s a lot of embedded CO2 in the production and transport of input materials that are not always factored into carbon-neutrality claims.” This, Dailey says, is a thriving area of research and debate that has gone all the way up to the United Nations.
In the event that a CCU product is made in a carbon neutral or carbon negative way, some products like carbon-captured Cola will cause us to “burp the carbon right back out,” Dailey notes. Others store it for much longer.
While it's done in a myriad of ways, CCU is divided into four umbrella categories: direct use (in the case of EOR), fermentation, chemical conversion, and mineralization. Each stores the carbon for a different amount of time.
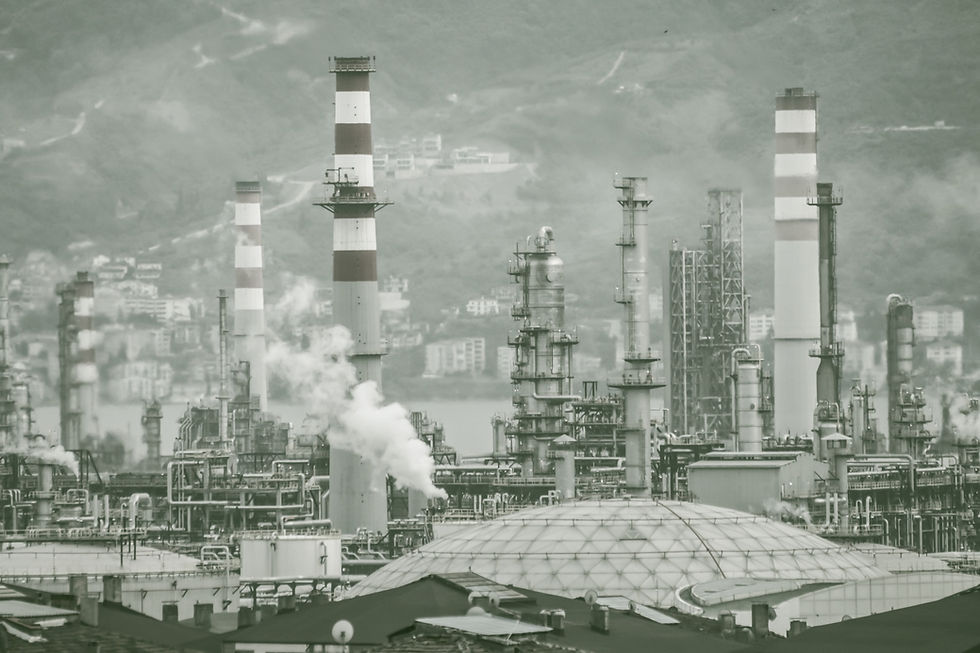
According to an analysis in Nature, carbon stored with EOR can last for years to centuries. Unlike EOR, products made with CCU undergo a conversion process.
In the first approach, fermentation, captured carbon lasts for months to decades. This process uses microbes to make biofuels like methanol, ethanol, and methane.
Through fermentation, carbon is put into juicy steaks and scallops by companies like AirProtein. Companies like Steelanol use waste carbon from steelmaking plants, turning it into bioethanol for glass, paper, or plastic. Fermentation is the same process used to make spirits like wine and beer, so it should come as no surprise that it’s also used to make the famous carbon vodka.
The next approach, chemical conversion, uses polymers, a staple for the global economy used in almost every area of modern living. This approach is what could allow the plastic industry to become a carbon-capture leader. As highlighted by the market research firm, IDTechEx, CO2-based polymers have potential in electronics, foam, mulch, and even biomedical and healthcare sectors.
While this approach takes up a small portion of the sector, a success story has been LanzaTech, which has established partnerships with major brands like Unilever, L'Oréal, On, Danone, Zara, and Lululemon to make captured carbon packaging items, shoes, and textiles. But, as Nature reports, the polymer approach keeps carbon contained for mere decades.
The last approach is mineralization. This process injects carbon into building materials. Many companies like CarbonCure, put this carbon into one of the most abundant materials on Earth: concrete.
Mixing carbon with cement, water, and sand creates a chemical reaction, turning carbon into a mineral. CarbonCure has a goal of removing 500 million tons of CO2 annually from the concrete industry by 2030. As reported by the company, last year, the technology avoided more than 75,000 tons of CO2, making a difference in the 2.7 billion tons of CO2 cement production causes a year.
Other startups like Terra CO2, are working to make the cement itself carbon-capturing. Of the CCU product list, mineralization stores carbon for the longest, ranging from decades to centuries.
However, because carbon sequestered in products is generally short-lived, it creates what Dailey refers to as a “tension” in the industry.
Mineralization and geologic storage keep carbon out of the atmosphere the longest. Thus, in some cases, products like concrete are advertised as a vessel to store carbon perpetually. Nevertheless, that so-called “forever” is on a human scale. Concrete, buildings, and sidewalks won’t necessarily last for millennia, she said. And while carbon in products like aquaculture fish food won’t last long, Dailey says “it’s an entirely different approach than sequestration.”
“There’s this tension from a climate impact point of view,” she says. “CCU products are not always good at keeping CO2 out of the atmosphere - they capture the CO2 but often re-release it via their products’ use. But the CCU industry is building a marketplace, so that people start to care about captured carbon, will pay for carbon, and help carbon removal companies scale down the cost, and that’s incredibly valuable.”
A lot of premium products like carbon clothes, accessories, and jewelry are luxury items, but it’s argued the exorbitant price doesn't diminish the need for them.
According to Dailey, “Because the technology for direct air capture and for a lot of carbon capture and utilization technologies is new, cutting edge, and expensive, there’s this need right now for buyers who are willing to pay a premium to help facilitate plants being built, to learn and scale the technology, and lower capture costs.” Even if they’re more expensive now, “we need more money and investment in this space so we can scale it and make it cheap.”
To Dailey, long-term geologic storage is the goal. But in the meantime, those carbon yoga pants and infused diamond rings might just help make full geologic storage a reality.
Comments